Vol. 7, No 16, p. 905-936 - 31 ago. 2020
Avaliação ambiental da produção de ferro gusa: revisão sistemática da literatura, bibliometria e patentes
Adriano Souza Leão
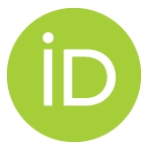
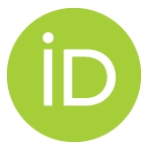
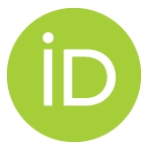
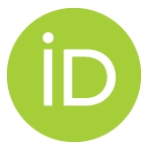
Resumo
A siderurgia de ferro e aço tem sofrido pressões e transformações determinantes nos últimos anos em função dos impactos ambientais gerados pelo setor. O ferro gusa, principal matéria-prima do aço, é produzido majoritariamente por meio de alto-fornos, uma tecnologia intensiva em consumo de energia, de recursos primários e, também, em emissão de gases de efeito estufa (GEE). Ao passo que o setor se vê pressionado com a escassez de matérias-primas e as repercussões da mudança climática, rotas tecnológicas em processo de desenvolvimento têm indicado uma potencial melhoria no desempenho ambiental do ciclo de vida do ferro gusa. A Avaliação de Ciclo de Vida (ACV) é um método que visa a estimar os impactos ambientais de produtos, serviços e processos, considerando desde a cadeia de suprimentos até a obtenção do produto final, bem como uso, e descarte final. Neste estudo, realizou-se uma revisão sistemática da literatura, análise bibliométrica e de patentes com o objetivo de levantar evidências e investigar as lacunas acerca dos aspectos ambientais da produção de ferro gusa, dos impactos do seu ciclo de vida e das reivindicações de inovação na siderurgia associadas às tecnologias "verdes". Verificou-se uma tendência de crescimento tanto em contribuições científicas quanto em registros de patente na última década, acentuada a partir de 2013. Os tópicos em destaque na discussão acadêmica abrangem desde inventários de fluxos no início da década, até o desempenho ambiental do processo produtivo, nos estudos mais recentes. O domínio tecnológico esteve focado na viabilização do reaproveitamento de resíduos, desenvolvimento de materiais alternativos e otimização de processo. Geograficamente, os países asiáticos, sobretudo a China, destacaram-se com a maior quantidade de contribuições acadêmicas e tecnológicas, embora países da Europa, das Américas e a Austrália também tenham se mobilizado ativamente. A convergência observada nesses resultados evidenciou a ocorrência de fenômenos análogos em diferentes perspectivas de produção de conhecimento: academia e indústria. Por fim, o tema revela ainda possuir potencial de aprofundamento, especialmente com respeito aos fornos em desenvolvimento.
Palavras-chave
Pensamento do Ciclo de Vida (PCV); Ecologia Industrial; Tecnologia emergente; Pegada de carbono;
Eficiência energética.
Abstract
Environmental assessment of pig iron production: Systematic review of literature, bibliometrics and patents.
The iron and steel industry has undergone significant pressures and transformations in recent years due to the
environmental impacts caused by the sector. Pig iron, the main raw material of steel, is predominantly produced by
blast furnaces, a technology that is intensive in energy use, primary resource consumption as well as greenhouse
gas (GHG) emission. While the sector is pressured by the scarcity of raw materials and the repercussions of climate
change, technological routes in development process have pointed to a potential for a better environmental
performance of the pig iron life cycle. The Life Cycle Assessment (LCA) is a method that aims to estimate the
environmental impacts of products, services and processes, considering from the supply chain to the final product,
as well as use, and final disposal. In this study, a systematic review of literature, bibliometric and patent
analysis were conducted to collect evidence and investigate the gaps concerning the environmental aspects of pig
iron production, the impacts of its life cycle and the claims of innovation in the steel industry associated with
"green" technologies. There has been an upward trend in both scientific contributions and patent
registrations over the past decade, intensified since 2013. The topics highlighted in the academic discussion range
from flow inventories at the beginning of the decade to the environmental performance of the manufacture process,
in the most recent studies. The technological domain was focused on the viability of waste reuse, development of
alternative materials and process optimization. Geographically, Asian countries, especially China, stood out with
the highest number of academic and technological contributions, although countries in Europe, the Americas and
Australia also have actively mobilized. The convergence observed in these results showed the occurrence of similar
phenomena in different perspectives of knowledge production: academia and industry. Finally, the theme reveals still
potential for further development, especially with regard to developing furnaces.
Keywords
Life Cycle Thinking (LCT); Industrial Ecology; Emerging technology; Carbon footprint; Energy efficiency.
DOI
10.21438/rbgas(2020)071629
Texto completo
PDF
Referências
ABNT - Associação Brasileira de Normas Técnicas. NBR ISO 14040: Gestão ambiental -
avaliação do ciclo de vida - princípios e estrutura. Rio de Janeiro: ABNT, 2006.
Arasto, A. Techno-economic evaluation of significant CO2 emission reductions in the iron and steel
industry with CCS. Finlândia: Aalto University. 2015. (Tese de doutorado). Disponível em:
<https://aaltodoc.aalto.fi/handle/123456789/18765>.
Acesso em: 29 jun. 2020.
Bahadir, M. E. Analysis of the environmental effects of international outsourcing: study of the iron casting
industry. Scientific Research and Essays, v. 11, n. 16, p. 160-173, 2016. https://doi.org/10.5897/SRE2016.6421
Bains, P.; Psarras, P.; Wilcox, J. CO2 capture from the industry sector. Progress in Energy and
Combustion Science, v. 63, p. 146-172, 2017. https://doi.org/10.1016/j.pecs.2017.07.001
Burchart-Korol, D. Fossil fuels consumption evaluation in blast furnace technology based on different life
cycle impact assessment methods. Anais do Metal2012, 21st International Conference on Metallurgy and
Materials, 2012. Disponível em: <http://metal2012.tanger.cz/files/proceedings/02/reports/137.pdf>.
Acesso em: 29 jun. 2020.
Burchart-Korol, D. Life cycle assessment of steel production in Poland: A case study. Journal of Cleaner
Production, v. 54, p. 235-243, 2013. https://doi.org/10.1016/j.jclepro.2013.04.031
CGEE - Centro de Gestão e Estudos Estratégicos. Siderurgia no Brasil: 2010-2025.
Brasília: CGEE, 2010. Disponível em: <https://www.cgee.org.br/documents/10195/734063/Siderurgia_no_Brasil__9567.pdf/893da7ee-8608-4251-adc1-10c2bf95b009?version=1.0>.
Acesso em: 29 jun. 2020.
Chen, S.; Fu, X.; Chu, M.; Liu, Z.; Tang, J. Life cycle assessment of the comprehensive utilisation of
vanadium titano-magnetite. Journal of Cleaner Production, v. 101, p. 122-128, 2015.
https://doi.org/10.1016/j.jclepro.2015.03.076
Choi, H. D. Hybrid life cycle assessment of steel production with carbon capture and storage.
Trondheim: Norwegian University of Science and Technology, 2013. (Dissertação de Mestrado).
Disponível em: <http://hdl.handle.net/11250/235312>.
Acesso em: 29 jun. 2020.
Chowdhury, J. I.; Hu, Y.; Haltas, I.; Balta-Ozkan, N.; Varga, L. Reducing industrial energy demand in the UK:
A review of energy efficiency technologies and energy saving potential in selected sectors. Renewable and
Sustainable Energy Reviews, v. 94, p. 1153-1178, 2018. https://doi.org/10.1016/j.rser.2018.06.040
Crossin, E. The greenhouse gas implications of using ground granulated blast furnace slag as a cement substitute.
Journal of Cleaner Production, v. 95, p. 101-108, 2015. https://doi.org/10.1016/j.jclepro.2015.02.082
Ding, X.; Li, H. Environmental benefit from blast furnace gas recycling in the integrated steelworks. Anais do
2011 International Conference on Computer Distributed Control and Intelligent Environmental Monitoring, IEEE,
p. 2091-2095, 2011. https://doi.org/10.1109/CDCIEM.2011.427
Fick, G.; Mirgaux, O.; Neau, P.; Patisson, F. Environmental assessment of biomass options for ironmaking. Anais
do Carbon Management Technology Conference, 2012. https://doi.org/10.7122/151138-MS
Fick, G.; Mirgaux, O.; Neau, P.; Patisson, F. Using biomass for pig iron production: A technical, environmental
and economical assessment. Waste and Biomass Valorization, v. 5, n. 1, p. 43-55, 2014. https://doi.org/10.1007/s12649-013-9223-1
Gielen, D.; Moriguchi, Y. Environmental strategy design for the Japanese iron and steel industry. 2001.
Disponível em: <https://www.gtap.agecon.purdue.edu/resources/download/461.pdf>.
Acesso em: 29 jun. 2020.
Gordon, Y.; Kumar, S.; Freislich, M.; Yaroshenko, Y. The modern technology of iron and steel production and
possible ways of their development introduction. Steel in Translation, v. 45, n. 9, p. 627-634, 2015.
https://doi.org/10.3103/S0967091215090077
Hasanbeigi, A.; Price, L.; Arens, M. Emerging energy-efficiency and carbon dioxide emissions-reduction
technologies for the iron and steel industry. Berkeley: Lawrence Berkeley National Laboratory, 2013.
(LBNL-6106E). Disponível em: <https://escholarship.org/uc/item/5sw966f9>.
Acesso em: 23 jan. 2020.
IBICT - Instituto Brasileiro de Informação em Ciência e Tecnologia. Guia geral para
avaliações do ciclo de vida orientações detalhadas. Brasília: IBICT,
2014. Disponível em: <http://acv.ibict.br/wp-content/uploads/2016/01/Manual-ILCD-com-capa.pdf>.
Acesso em: 23 jan. 2020.
IEPDS - International Environmental Product Declaration System. Product category rule for basic iron or
steel products & special steels, except construction steel products: Product category rules according
to ISO 14025. Estocolmo: IEPDS, 2015. Disponível em: <https://www.environdec.com/PCR/Detail/?Pcr=10372>.
Acesso em: 23 jan. 2020.
IIMA - International Iron Metallics Association. Pig iron: Overview. Burnham: IIMA, 2018. Disponível
em: <https://www.metallics.org/pig-iron.html>.
Acesso em: 23 jan. 2020.
Jahanshahi, S.; Mathieson, J. G.; Somerville, M. A.; Haque, N.; Norgate, T. E.; Deev, A.; Zulli, P. Development of
low-emission integrated steelmaking process. Journal of Sustainable Metallurgy, v. 1, n. 1, p. 94-114, 2015.
https://doi.org/10.1007/s40831-015-0008-6
Kuramochi, T. CO2 capture in industries and distributed systems: Possibilities and limitations.
Utrecht: Utrecht University, 2011. (Tese de doutorado). Disponível em: <https://dspace.library.uu.nl/handle/1874/205115>.
Acesso em: 29 jun. 2020.
Lv, W.; Sun, Z.; Su, Z. Life cycle energy consumption and greenhouse gas emissions of iron pelletizing process in
China, a case study. Journal of Cleaner Production, v. 233, p. 1314-1321, 2019. https://doi.org/10.1016/j.jclepro.2019.06.180
Ma, F.; Eneji, A. E.; Wu, Y. An evaluation of input-output value for sustainability in a Chinese Steel Production
System Based on Emergy Analysis. Sustainability, v. 10, n. 12, 4749, 2018. https://doi.org/10.3390/su10124749
Miah, K. Use of waste resources in the sinter plant in ironmaking. Sheffield: University of Sheffield, 2018.
(Tese de doutorado). Disponível em: <http://etheses.whiterose.ac.uk/id/eprint/21560>.
Acesso em: 29 jun. 2020.
MME - Ministério de Minas e Energia. Anuário estatístico do setor metalúrgico.
Brasília: MME, 2018. Disponível em: <http://www.mme.gov.br/documents/1138775/1732813/ANUÁRIO+METALÚRGICO+2018.pdf/442af830-a9c6-4e75-bbc9-fef4b0f81300>.
Acesso em: 23 jan. 2020.
Mousa, E.; Wang, C.; Riesbeck, J.; Larsson, M. Biomass applications in iron and steel industry: An overview of
challenges and opportunities. Renewable and Sustainable Energy Reviews, v. 65, p. 1247-1266, 2016.
https://doi.org/10.1016/j.rser.2016.07.061
Norgate, T.; Langberg, D. Environmental and economic aspects of charcoal use in steelmaking. ISIJ international,
v. 49, n. 4, p. 587-595, 2009. https://doi.org/10.2355/isijinternational.49.587
Olmez, G. M.; Dilek, F. B.; Karanfil, T.; Yetis, U. The environmental impacts of iron and steel industry: A life cycle
assessment study. Journal of Cleaner Production, v. 130, p. 195-201, 2016. https://doi.org/10.1016/j.jclepro.2015.09.139
Pan, H.; Zhang, X.; Wu, J.; Zhang, Y.; Lin, L.; Yang, G.; Deng, S.; Li, L.; Yu, X.; Qi, H.; Peng, H. Sustainability
evaluation of a steel production system in China based on emergy. Journal of Cleaner Production, v. 112,
p. 1498-1509, 2016. https://doi.org/10.1016/j.jclepro.2015.05.019
Ramos, C. G. Drivers and barriers to industrial energy efficiency and climate change mitigation in Mexico:
The case of the iron and steel industry. East Anglia: University of East Anglia, 2011. (Tese de doutorado).
Disponível em: <https://ueaeprints.uea.ac.uk/id/eprint/32708/1/2011CastilloRamosGPhD-4.pdf>.
Acesso em: 29 jun. 2020.
Reuter, M. A.; Van Schaik, A.; Gediga, J. Simulation-based design for resource efficiency of metal production and
recycling systems: Cases-copper production and recycling, e-waste (LED lamps) and nickel pig iron. The International
Journal of Life Cycle Assessment, v. 20, n. 5, p. 671-693, 2015. https://doi.org/10.1007/s11367-015-0860-4
Rojas-Cardenas, J. C.; Hasanbeigi, A.; Sheinbaum-Pardo, C.; Price, L. Energy efficiency in the Mexican iron and
steel industry from an international perspective. Journal of Cleaner Production, v. 158, p. 335-348, 2017.
https://doi.org/10.1016/j.jclepro.2017.04.092
Ryman, C. On the use of process integration methods: Evaluation of energy and CO2 emission
strategies in blast furnace ironmaking and oxygen steelmaking. Luleå: Luleå University of
Technology. 2007. (Tese de doutorado). Disponível em: <https://www.diva-portal.org/smash/record.jsf?pid=diva2:999079&dswid=-4349>.
Acesso em: 29 jun. 2020.
Saade, M. R. M. Influência da alocação de impactos na indústria siderúrgica
sobre a avaliação de ciclo de vida de cimentos. Campinas: UNICAMP, 2013. (Dissertação
de mestrado). Disponível em: <http://repositorio.unicamp.br/handle/REPOSIP/258517.
Acesso em: 29 jun. 2020.
Saade, M. R. M.; Silva, M. G.; Gomes, V. Appropriateness of environmental impact distribution methods to model blast
furnace slag recycling in cement making. Resources, Conservation and Recycling, v. 99, p. 40-47, 2015.
https://doi.org/10.1016/j.resconrec.2015.03.011
Sablowski, A. R. M. Balanço de materiais na gestão ambiental da cadeia produtiva do carvão
vegetal para produção de ferro gusa em Minas Gerais. Brasília: Universidade de Brasília,
2008. (Tese de doutorado). Disponível em: <https://ri.ufs.br/handle/riufs/811.
Acesso em: 29 jun. 2020.
Shatokha, V. Trends and factors of sustainable development: Best available and breakthrough. In: Shatokha, V.;
Stalinskiy, D.; Coole, T.; De Lepeleer, G.; Karpash, M.; Kruhlenko, L.; Petrenko, A.; Saey, P.; Shvets, I.
Environmentally sustainable industrial development. Dnipro: Hetes, 2017. p. 140-164. Disponível
em: <http://hetes.com.ua/wp-content/uploads/2017/10/common_book_HETES.pdf>.
Acesso em: 29 jun. 2020.
Shatokha, V.; Tymoshenko, S.; Semenko, S. Trends and factors of sustainable development: Iron and steelmaking.
In: Shatokha, V.; Stalinskiy, D.; Coole, T.; De Lepeleer, G.; Karpash, M.; Kruhlenko, L.; Petrenko, X. A.;
Saey, P.; Shvets, I. Environmentally sustainable industrial development. Dnipro: Hetes, 2017. p. 56-78.
Disponível em: http://hetes.com.ua/wp-content/uploads/2017/10/common_book_HETES.pdf>.
Acesso em: 29 jun. 2020.
Smil, V. Modern ironmaking and steelmaking: Furnaces, processes, and casting. In: Smil, V. Still the Iron
Age: Iron and steel in the Modern World. Amsterdam: Elsevier, 2016. p. 87-114. <https://doi.org/10.1016/B978-0-12-804233-5.00005-1
Suopajärvi, H.; Pongrácz, E.; Fabritius, T. Bioreducer use in Finnish blast furnace ironmaking:
Analysis of CO2 emission reduction potential and mitigation cost. Applied Energy, v. 124,
p. 82-93, 2014. https://doi.org/10.1016/j.apenergy.2014.03.008
Suopajärvi, H.; Kemppainen, A.; Haapakangas, J.; Fabritius, T. Extensive review of the opportunities to
use biomass-based fuels in iron and steelmaking processes. Journal of Cleaner Production, v. 148,
p. 709-734, 2017. https://doi.org/10.1016/j.jclepro.2017.02.029
Suopajärvi, H.; Umeki, K.; Mousa, E.; Hedayati, A.; Romar, H.; Kemppainen, A. Chuan, W.; Aekjuthon, P.;
Sari, T.; Nicklas, N.; Alf, A.; Marcus, Ö.; Ulla, L.; Timo, F. Use of biomass in integrated steelmaking:
Status quo, future needs and comparison to other low-CO2 steel production technologies.
Applied Energy, v. 213, p. 384-407, 2018. https://doi.org/10.1016/j.apenergy.2018.01.060
Vadenbo, C. O.; Boesch, M. E.; Hellweg, S. Life cycle assessment model for the use of alternative resources
in ironmaking. Journal of Industrial Ecology, v. 17, n. 3, p. 363-374, 2013. https://doi.org/10.1111/j.1530-9290.2012.00543.x
Wickboldt, L. A.; Moreira, J. D. A. P.; Silva, M. D. R. D.; Araújo, J. D. S.; Silva, J. V. D.; Pereira,
J. A. Responsabilidade ambiental ou greenwash: uma análise da evidenciação ambiental das
maiores empresas brasileiras. Revista Brasileira de Gestão Ambiental e Sustentabilidade, v. 5,
n. 11, p. 897-910, 2018. https://doi.org/10.21438/rbgas.051108
WSA - World Steel Association. Steel statistical yearbook 2018. Bruxelas: World Steel Association. 2018.
Disponível em: <https://www.worldsteel.org/en/dam/jcr:e5a8eda5-4b46-4892-856b-00908b5ab492/SSY_2018.pdf>.
Acesso em: 23 jan. 2020.
Wu, J.; Wang, R.; Pu, G.; Qi, H. Integrated assessment of exergy, energy and carbon dioxide emissions in an
iron and steel industrial network. Applied Energy, v. 183, p. 430-444, 2016. https://doi.org/10.1016/j.apenergy.2016.08.192
ISSN 2359-1412